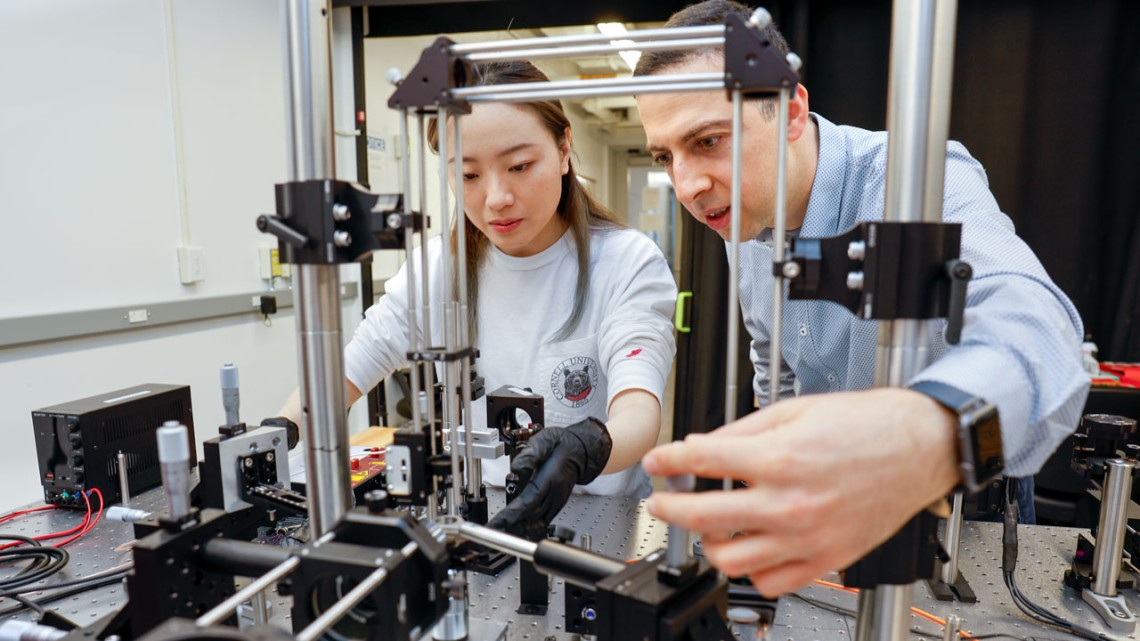
Assistant professor Mostafa Hassani (right) and doctoral student Qi Tang work with a custom-built platform to launch supersonic microprojectiles and study how high-speed metallic collisions form solid-state bonds.
Supersonic microprojectiles reveal new insights into metal bonding
By Syl Kacapyr
Using a custom-built machine to launch microprojectiles at supersonic speeds, Cornell researchers have uncovered new details about how high-speed metallic collisions can form strong, durable atomic bonds, offering insights that could enhance 3D printing and other manufacturing techniques.
When a microparticle collides with a metallic substrate at supersonic speed, a process known as solid-state bonding can occur, in which two metals are joined at the atomic level. While the conditions for bonding are relatively well understood, the microstructure and the material properties formed in these high-speed collisions have remained mostly uncharacterized.
A study published Nov. 7 in Nature Communications details at the micrometer scale the strength and gradient of atomic bonds across supersonic impact interfaces, and presents a framework for predicting the outcomes of solid-state bonding.
“This marks a paradigm shift in our understanding of the process-microstructure-property relationships in impact-induced bonding,” said senior author Mostafa Hassani, assistant professor in the Sibley School of Mechanical and Aerospace Engineering and in the Department of Materials Science and Engineering. “These findings will enable reliable and performance-oriented design of surface modification, repair and additive manufacturing technologies that rely on supersonic impact bonding.”
Supersonic 3D printing, also known as “cold spray,” enables materials production without heating or melting, resulting in superior mechanical properties compared to conventional manufacturing processes. These advantages make it particularly well-suited for structural applications in aerospace and energy.
To create the solid-state bonding, the researchers built a laser-induced launch platform capable of precisely accelerating micrometer-sized aluminum particles to more than 2,200 miles-per-hour toward an aluminum substrate. Following the impact, micromechanical tensile testing was conducted using a scanning electron microscope to directly measure the bond strength at different locations across the impact interface.
The study revealed that the bond strength is not uniform, but varies significantly from the center of the impact to the edges. In particular, a weak bond exists at the center of the impact, followed by a rapid twofold increase in bond strength that eventually plateaus toward the outer edges.
“A key finding is that the form of the native oxide at the interface – whether layers, particles or debris – dictates the level of bond strength locally,” Hassani said. “Specifically, regions with scattered oxide debris exhibited much stronger bonds than regions where the oxide layer remained mostly intact.”
To explain the variation in bond strength, the researchers developed a predictive model that accounts for two primary factors: contact pressure and surface exposure. As a microparticle impacts the substrate, the shear forces caused by the collision fracture the oxide layer, exposing more metal surface. Simultaneously, the pressure generated by the impact forces this newly exposed surface into atomic-scale proximity, creating a strong metallic bond.
“This understanding opens up new possibilities for tailoring interfacial properties and designing impact conditions – such as particle and substrate materials, particle size, velocity and temperature – to enhance bonding and interfacial strength,” said Qi Tang, doctoral student and lead author of the study. “It also offers insights for preventing bonding. For example, engineering surface material structures to prevent contamination from supersonic space dust impact-bonding on spacecraft shields or telescope lenses.”
The research was supported by the National Science Foundation and the Japan Science and Technology Agency.
Syl Kacapyr is associate director of marketing and communications for Cornell Engineering.
Media Contact
Get Cornell news delivered right to your inbox.
Subscribe